Clams
All reef aquarists
are familiar with the beautiful Tridacnid clams found in the
shallow waters of coral reefs throughout the Pacific. Tridacnids,
however, are very unusual clams; they have symbiotic zooxanthellae
and they also have an internal anatomy that is oddly oriented
relative to their shells. In this column I will discuss some
aspects of basic clam biology and some of the other species
that are occasionally available in the aquarium trade. Clams
or bivalves are mollusks and members of the Class Bivalvia
of the Phylum Mollusca. These are animals with a consistent
morphology and physiology. Except for a few structures, the
basic internal anatomy and structures are rather similar throughout
the class.
Two Shells
Although the name "clam"
does not at all clearly indicate what obvious and completely
diagnostic features these animals possess, the term "bivalve"
suggests these animals' most evident primary structures, namely,
the two shells, one on each side of the body. Although it
may not be obvious to many people, the term "bivalve"
is very descriptive. The term "valve" is an older,
somewhat archaic English term for "shell," so "bivalve"
is a noun meaning "two shells." The full name of
the group in question is then, "bivalved mollusks,"
but this is generally just shortened to "bivalves,"
and it is the name used to describe the group of animals we
more commonly call "clams." Using the same root
terminology, a name occasionally used for snails is "univalved
mollusks," or mollusks with only one shell.
The property of having two shells is, interestingly enough,
not unique to clams within the mollusks. All of the individuals
within one small group of tiny snails also possess two shells.
These peculiar snails in the genera Berthelinia
and Julia
occasionally show up in aquaria (Shimek, 2004). They are odd
little animals that are related to the lettuce slugs that
prey on Caulerpa. They have a pair of brilliant green
shells that are structurally quite similar to those found
on clams. As a matter of fact, these animals' shells were
collected as fossils
in the late nineteenth century, well before the living animals
were discovered, and they were scientifically described and
named as a type of fossil clam. After the discovery of the
living bivalved snails in the late 1940s, that error was corrected
and the animals were scientifically rediscribed as the only
bivalved gastropods. Except for these few, rather odd snails,
no living mollusks other than bivalves have two shells.
One other group of animals is "bivalved" and may
be confused with the clams. In fact, these animals, the lamp
shells or brachiopods,
may look quite "clammy." There are two quite distinct
types of brachiopods, both of which are bivalved. Specimens
of one type, the so-called "articulate brachiopods,"
are occasionally found as hitchhikers in aquaria attached
to live rock. The shells of these animals differ from clams
in that they are never symmetrical and one of them always
has a hole for a fleshy stalk that extends through the shell
that is used for fastening the animal securely to the substrate.
This stalk is stubby, just long enough to lift the animal
off the substrate and allow it to pivot. Many types of clams
live fastened to the rock, but never by a stalk that allows
them to pivot. Examples of a second brachiopod type, called
"inarticulate brachiopods," may occasionally be
purchased by aquarists. These animals live in sand and have
symmetrical, somewhat clam-like shells; however, a long fleshy
stalk protrudes from the back of the shells where they are
connected together. The stalk extends down into the sediment,
fastening the animal in place. A clam may extend its foot
down into the sediment, but if it does, that foot extends
from the gaping region of the clam's shells, normally opposite
the side where the shells are connected. The clam's foot is
withdrawn completely within the shells when the animal closes
its shells, while the stalk of the inarticulate brachiopod
remains outside its shells at all times.
What's on the Mantle?
A clam is an animal
with a small body enclosed within a space created by an external
shell on each side of its body. The shells generally connect
or are hinged together at the top (or "anatomically"
dorsal apex) of the body. Differences in the position and
structure of the connection between the shells are used to
delineate some of the clam subgroupings. A ligament made of
a resilient rubbery protein holds the shells together, and
acts like a spring pushing the shells open. This means that
a clam must actively contract muscles to close its shells.
When these muscles relax, the shell opens passively. If you
are wondering about the condition of a clam in your tank,
an easy first determination of its health may be made by gently
touching the shell, or the tissue layer around the shells.
If the animal contracts rapidly, it is probably healthy. If
it contracts slowly or feebly, it may be in trouble. If it
doesn't contract at all, it is dead.
The shells are secreted by a layer of tissue on the outside
of each side of the body called the mantle. This mantle lines
the shell extending down each side of the body from the dorsal
region near the hinge between the shells to the shells' outer
edges. Deposition of shell material on the inner shell surface
by the underlying mantle thickens the shell. This deposition
continues throughout the clam's life. If the shell is broken,
but the animal is not killed, subsequent deposition of shell
material on the shell's inner surface will heal the fracture.
The shells grow by deposition of shell material on their outer
edges. Here, the deposition is done by a region near the mantle's
outer edge. Fibers extend into the shell from just inside
the mantle's outer edges and fasten the mantle to the shell.
The mantle's outermost region may extend beyond the shell
and be elaborated into sensory tentacles or other sensory
structures, such as the eyes found in scallops. These structures
generally are thought to function in detecting potential predators.
Most bivalves can't move either rapidly or far, and therefore
avoid predation by withdrawing into their shells and closing
them tightly, so it is important for them to be able to detect
the approach of predators.
In many clams, the mantle's posterior edges may fuse together
where they meet and form tubes or siphons. The siphons guide
incurrent (entering) and excurrent (exiting) streams of water.
Clams are basically filtering pumps, and the direction and
separation of water currents is vitally important to them.
These siphons may be quite long. In the geoduc (pronounced
"gooey
duck"), Panopea abrupta, of the Pacific coast
of North America, the siphons may extend a meter or more.
Inside the shells, the body is suspended directly below the
hinge and hangs in the cavity created and enclosed by the
shells. Because this cavity is lined by the mantle, it is
called the "mantle cavity" and it surrounds the
body on all sides. Relative to the size of the body, this
cavity is often quite spacious, and is a "hydrodynamic"
space through which water is drawn, directed, filtered and
used to flush and clean the animal.
Inside the Envelope
Internally, most clams have a consistent,
relatively simple and straightforward morphology. Much of
this simplicity is due to reductions from the standard molluscan
body plan. Normally, mollusks have good heads and sensory
organs; however in bivalves, except for the mouth and lips,
all normal head structures, including the brain, are absent.
Presumably if all you do is sit in one spot and filter water,
you don't need much in the way of a brain - and bivalves don't
have one. The rest of their nervous system is not terribly
sophisticated, either. The major components of their nervous
system are a few pairs of small and simple ganglia, or nerve
cell aggregations, one on each side of the animal. There are
typically three major pairs of ganglia. One pair, located
near the mouth, seems to control feeding. Another pair in
the visceral mass probably controls digestive and reproductive
activities, and a pair in the foot likely controls any locomotion.
As one might expect, the array of sensory organs is decidedly
limited. Most bivalves have a few sensory tentacles around
the mantle or siphon edges. Many have a statocyst, or balance
organ, that allows them to determine their orientation in
the sediments. A few have enervated pigmented spots, termed
"eye spots," that seem to be photosensory. These
are generally located on the mantle but in a few clams they
may be located on the siphons or viscera. The most sophisticated
and complex sensory structures found in any of the clams are
the eyes of scallops. These eyes present a paradox; although
they are quite capable of forming an image, the animal simply
does not have sufficient nerve cells to process or "see"
such an image. Instead, these eyes appear to function as sophisticated
shadow receptors, signaling when light changes rapidly. This
would happen, for example, when a predatory fish swimming
over the scallop casts a shadow upon it. This can trigger
the scallop to swim by clapping its valves rapidly together;
this causes a jet of water to exit from around the hinge,
thereby propelling the animal away from harm.
Figure 1. This diagram of a typical lamellibranch clam,
on the half shell, shows some of the major body parts on one
side of the body. The animal is bilaterally symmetrical, and
gills and labial palps would be found on the other side of
the body as well. The foot extends from between the gills,
which are large enough to cover it. The water flow is shown
in blue entering through the lower incurrent siphon, passing
through the gills and out the excurrent siphon. The yellow
lines show the fate of food captured on the gill as it moves
to the mouth in mucus in a food groove. The rest of the animal's
body is shown in green.
Figure 2. This shows the internal organs of a typical
bivalve as in Figure 1. The orange digestive gland and its
connection to the stomach are shown. The gonad is shown in
green, and it and the digestive gland ramify together in the
foot and surround the stomach. The kidney is shown as an orange
hatched region over the heart. On each side the auricle receives
blood from the gill on that side. The singe ventricle surrounds
the intestine and typically pumps blood forward through a
vessel to tissue spaces where it percolates through the rest
of the body.
Figure 3. Left: Scallops are common swimming
bivalves found in all seas. They swim by "clapping"
their valves together. This forces a jet of water out the
back by the "ears." Right: The swimming is
random and non-directional. They are triggered to swim by
stimuli sent by their eyes, which are visible as dots near
the edge of the shells.
Getting From Here to There
at a Clam's Pace
In contrast to the
swimming ability seen in scallops, most clams cannot move
much. Many of them have a foot which can extend out of the
shell. While a few forms, such as razor clams, can burrow
very rapidly, most sediment dwelling clams can't burrow rapidly
at all. Other species, such as the large geoducs, are immobile
as adults. As juveniles they grow and burrow deep into the
sediments, but once they reach adulthood, they live in their
hole in the ground, as much as two feet or more below the
sediment's surface. When disturbed, they may pull their siphon
rapidly down into the sediment, but the rest of the animal's
body doesn't move.
In some clams the foot may also fasten the animal to the
substrate. The foot of these animals contains a gland which
secretes a material called "byssus;" thus the gland
is called the byssal gland. Byssus generally consists of proteinaceous
strands. The animal secretes these as liquid strands that
harden to the substrate and then are extended up into the
water as threads or strings. The animal then physically holds
on to them. These strands are quite strong, capable of holding
a mussel in place in pounding surf, for example. However,
the animal may let go of them at will, and this property allows
animals such as mussels, which are usually thought of as stationary,
to be quite mobile. They move by sequentially depositing and
then releasing their byssus. If you have a mussel in your
aquarium, it is easy to follow where it has moved by following
the trails of byssus that remain attached to the substrate.
Incidentally, the rapidly swimming scallops also may secrete
byssal attachments. When the scallop wants to swim, it simply
lets go of the byssus and swims away.
Visceral Reactions
Being basically
designed to sit in one place and convert particulate organic
material into clam tissue, the internal structures of clams
are not particularly complex. The gut is a good example of
this simplicity. The mouth leads to an esophagus that may
be, relative to the size of the animal, quite long. This,
in turn, empties into a relatively large stomach. A digestive
gland is found on either side of the stomach. These glands,
sometimes inappropriately called "livers," are the
site where most digestion occurs. A fairly short intestine
leads out of the posterior end of the stomach and terminates
in an anus near the opening of the excurrent siphon.
This basic simplicity aside, however, the processing and
digestion of foods is quite unlike that seen in most animals.
The typical processes of digestion that the reader may be
familiar with in vertebrates are wholly absent, and present
a good example of how different animals attack the same fundamental
problem of digestion of food. Clams are generally adapted
to eat very fine particulate food, and are hence called "microphagous"
feeders. Probably the most structurally complicated part of
any bivalve is its stomach. This organ is superbly adapted
to assist in the digestion of very fine particulate material.
In the case of most bivalves, this material is phytoplankton,
microalgal cells suspended in the water. The filtered particulate
food is collected from the gills onto streams of mucus that
enter the mouth, where they are compacted into a single strand
that is swallowed. This mucus strand or string extends through
the esophagus into the stomach, which is a bag-like structure
with several peculiarities. Near its anterior end is located
a hardened cuticular plate called the "gastric shield."
This plate is made of protein and chitin and forms a roughened,
rasp-like surface. At the stomach's opposite end is a pouch.
This pouch is secretory and produces a rod, called a "style,"
made of a mass of hardened mucus and digestive enzymes. This
rod may be quite long, often two to three times the length
of the shell, and the pouch that holds and produces it may
be folded around the stomach. The rod is flexible and bends
to follow the shape of the pouch. The rod is spun by beating
cilia in the pouch and may rotate at speeds up to about 600
revolutions per minute.
 |
Figure 4. A diagrammatic representation of a typical
bivalve stomach showing the parts discussed above.
The mucus string coming from the mouth is wrapped around
the spinning rod and pulled into the stomach much as rope
around a windlass. Secretion adds material to the rod rapidly
as it spins, but it doesn't increase in length. Instead, the
free end is pressed against the cuticular rasp and ground
to pieces, thoroughly mixing the particulate food with digestive
enzymes from the rod. The resulting slurry drops to the bottom
of the stomach where the stomach's pH changes, causing the
mucus to dissolve. The floor of the stomach is covered with
corrugations, grooves and ridges, all covered with microscopic
beating cilia. These cilia set up currents in this "sorting
region" and particles of the appropriate size and density
are conveyed by cilia to the digestive gland openings on either
side of the stomach. Inedible material, such as mineral grains,
or particles that are too large, is moved to the far end of
the stomach. In this area, the pH becomes more alkaline, and
the mucus again solidifies. Here, the indigestible food and
mucus are compacted together into another mucus strand that
will pass out of the intestine in a groove called the typhlosole.
Food particles that enter the digestive gland are ingested
by individual cells and digested internally. Unlike the condition
seen in humans or even some other mollusks, food is not digested
in a slurry of digestive juices within the gut cavity, but
rather internally within the individuals cells of the digestive
gland. Indigestible food residue is conveyed out of the gland
and added to the mucus strand which will become feces. Nutrients
are released from the digestive gland cells into the clam's
blood.
Circulation
Clams follow the typical molluscan
circulatory pattern and lack capillaries. Blood is pumped
out of the heart and goes through a few large vessels to the
tissues where it exits the arteries and enters large spaces
where it passes over the cells of the various organs. It then
collects into large internal spaces called lacunae from which
it passes through the kidney and gills, ultimately returning
to the heart to complete the circuit. The heart has two auricles,
one on either side, receiving oxygenated blood from the gills.
These auricles pump blood into the single ventricle which
then pumps blood to the body. An odd peculiarity of the bivalve
circulatory system is that the ventricle surrounds the intestine.
Consequently, it may be said, correctly, that the gut goes
through the heart.
The Next Generation
All clams reproduce in a relatively
similar manner. Their reproductive system is simple, consisting
of gonads and simple pores to release the gametes into the
mantle cavity. The gonads are highly branched and their branches
intertwine with those of the digestive gland. Both of these,
in turn, extend down into the foot, as do loops of the intestine.
The testes are typically bright white, while the ovaries are
often intensely colored, orange, green or red, by the yolk
that is found in the eggs. The gonads are basically a collection
of tubules joining to form the gonoducts. These empty out
of the gonopores located near the anus and release eggs or
sperm into the excurrent water current. The strong water flow
carries them out of the mantle cavity. Fertilization generally
occurs in the sea. The embryos typically develop rapidly into
small swimming and feeding larvae that develop two tiny little
shells. These settle out of the plankton in the appropriate
habitat and take up life as juveniles. In a few bivalve species,
the females retain the eggs in the mantle cavity where fertilization
occurs. The larvae develop in the mantle cavity and are finally
expelled as small juveniles, which have bypassed the planktonic
stage altogether. Most bivalves have separate sexes, but hermaphroditism
is common.
Most shallow-water bivalves are short-lived, having life
expectancies of probably less than ten years. Some, however,
live much longer. Geoducs from North America's Pacific Northwest
coast have
been documented with ages of 150 years, and the large
tridacnids may live a similar span. Deep-sea bivalves may
live much longer still, perhaps many centuries. Some of these
latter animals are predatory and have been documented to take
over a year to eat a single prey item (see, for example, Allen,
1983; Allen and Morgan, 1981).
Bivalve Diversity
Clams may be subdivided into different
groups based upon their gill morphology. There are three fundamentally
and structurally different types of gills, hence there are
three basic types of clams. These are generally called the
protobranchs, lamellibranchs and septibranchs. The root word
for gill in Latin is "branch," and these names mean,
respectively, "first gill," "flap or layer
gill" and "shelf gill." We can't see the gills
from outside the shells, but fortunately the basic shell shapes
often correlate with gill structure, thus allowing us to determine
which type of clam we have in our systems. In all bivalves,
the gills are the source of water currents both going into,
and out of, the shell.
The name protobranch means first gills, and these animals
are presumed to be similar to the ancestral clams. The first
bivalved mollusk fossils are found in rocks over 400 million
years old, and these tiny shells do bear a passing resemblance
to some living protobranchs, so maybe this is not too far
off the mark. Protobranchs have small gills and large extended
lips referred to as "labial palps." Their gills
have no feeding function and are only respiratory. These typically
small animals often live in muddy areas and use their palps
to suck up mud, which they ingest. They feed on small particulate
matter in the mud, such as detritus, bacterial aggregations,
and fecal pellets. They also may eat the mineral sediment
by digesting the bacteria it contains. Protobranchs are often
capable of moving rapidly through sediments. I know of no
protobranchs that are kept in the aquarium trade, but they
possibly could be kept in a reef aquarium with a well-developed
sand bed.
 |
Figure 4. The protobranch bivalve Yoldia scissurata
in sediment. It feeds by extending its labial palps
out of its shell and using them to ingest sediments.
|
Figure 5. A specimen of Yoldia scissurata
dissected by removing its left shell. The front of the
animal is to the left. Note the small gill and the relatively
large labial palp.
|
The majority of bivalves are lamellibranchs, and have very
small labial palps and very large gills. Although there are
several different types of lamellibranch gills, they all function
as both respiratory and filter-feeding organs. The gills are
bathed in mucus and water is pumped through them, collecting
small plankton in the mucus. The mucus is conveyed to the
mouth by a series of food grooves where it is eaten and forms
the mucous strand described above. Most of the larger lamellibranch
clams have limited mobility, although the smaller ones often
do move quite well. All of the clams normally seen in reef
tanks are lamellibranchs including ark shells, flame scallops,
oysters, and tridacnids. Except for the tridacnids, these
species are difficult to keep in reef aquaria as very little
phytoplankton is available for them to eat. Typically, they
live for a few months, while they exhaust their energy reserves
and then die. These animals should not be purchased unless
you know that you have enough phytoplankton in your system
to ensure their survival.
Figure 6. A lamellibranch clam dissected by removal
of its left valve. Note the relative sizes
of the gill and the tiny labial palp compared to those in
the protobranch. The gills act as
respiratory organs as well as feeding organs.
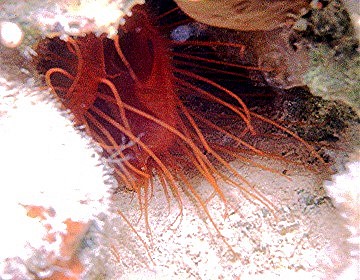 |
Figure 7. Although commonly kept, for
a while, by many aquarists, flame scallops, Lima
spp., such as this one photographed in the Caribbean,
have a dismal survival record in aquaria. They generally
starve after a few months. Only in aquaria where phytoplankton
is highly dosed, or whose water contains a lot of particulate
material, will these beautiful animals survive. Their
long sensory tentacles are extensions from the mantle's
edge.
|
The septibranchs are among the most bizarre clams and, probably
because of that, are my personal favorites. These animals'
gills are reduced to a muscular shelf or septum that extends
across the body. This siphon has "flapper" valves
so that when it contracts, water is pulled into the body.
They are found in sediments with the tip of their siphon just
at the sediment's surface, where they wait for a small crustacean
to encounter the siphon. When this occurs, the septum contracts,
and the bug is pulled into the mantle cavity and eaten. It
is mashed up and digested in the stomach. All septibranchs
are predatory. These animals are not seen in the aquarium
hobby, but should do well in most systems containing a well-developed
crustacean fauna. They are relatively uncommon in shallow
water, but are abundant in the very deep waters of the abyss.
Figure 8. Cuspidaria, a septibranch clam. Septibranchs
are often called "dipper shells"
as their calcareous siphon gives them the appearance of an
old fashioned dipper.
Bivalves in the form of tridacnids make beautiful and interesting
attractions in our reefs. Other clams may be equally attractive
but presently are difficult to keep. When we learn how to
consistently provide a phytoplankton food source most of these
clams will become attractive additions to our artificial ecosystems.
Figure 9. Siphons belonging to rock-boring clams living
inside live
rock are commonly found in reef aquaria.
|