As time goes by,
most reef aquarists will encounter a solid precipitate of
some sort in conjunction with their reef aquarium. Often these
are undesirable particles that cloud the water, coat the glass
or clog pumps. Others are more benign and disappear shortly
after forming. This article describes a variety of different
precipitates that aquarists encounter, and details what they
are, how and why they form, how to tell the different types
apart, whether they are a concern and how to avoid them.
The article is broken up into sections. The first ten sections
discuss different precipitates, while the latter seven discuss
the detailed information supporting what the precipitates
are and what factors contribute to their formation (such as
pH, temperature, etc.).
Contents:
Solid Residues Remaining After
Preparing Artificial Seawater
Most salt mixes leave behind a solid
residue when dissolved, although the extent to which this
occurs varies from brand to brand. I use Instant Ocean and
rarely clean out the 44-gallon trashcan that I mix it in,
so a significant residue builds up over time (Figure 1). In
preparation for this article I removed some of this solid
material, and found that it could be almost completely dissolved
in hydrochloric acid with lots of bubbling. This demonstrates
that these solids were probably calcium carbonate (CaCO3),
perhaps also containing magnesium.
Pure magnesium carbonate is undersaturated in seawater (which
is detailed in later sections of this article)
1 and should dissolve in marine systems,
so it isn't likely to be the precipitated material, although
there may be significant magnesium in the calcium carbonate.
Figure 1. The residue on the bottom of the plastic
trash can that I use to mix Instant Ocean. I rarely
clean it out. The solid is most likely calcium carbonate.
|
Based on the fact that the material exists as sheets that
clearly did not arrive in the mix (as opposed to a fine powder
which might have), I conclude that at least a significant
fraction of this residue formed in the barrel. I cannot, however,
rule out the possibility that some solid calcium or magnesium
carbonate may have existed in the salt mix and was cemented
together by additional precipitation of calcium carbonate
during dissolution or storage.
When salt mixes are dissolved, there exist local regions
where the salt concentration is very high. In those local
regions, the calcium
and alkalinity
must also be very high. In fact, as seawater is concentrated
by evaporation, there is a well-established series of minerals
that precipitate as the salinity increases. In this series,
calcium and magnesium carbonate are the first to precipitate,
appearing at a specific gravity of about 1.140, which is about
a 50% solution of salt in water.1
Such conditions may well exist on the bottom of a saltwater
reservoir as the salt is dissolving.
With some mixes (but not the Instant Ocean that I use), the
initial pH on dissolution may be very high (pH 8.5-9 +).
As shown in detail later in this article, pH can play a dominant
role in determining the rate of calcium carbonate precipitation,
and such a high pH would make it more likely to precipitate.
It has been suggested by some aquarists that some salt mixes
may contain anti-caking agents, such as clays. I do not know
if this is true, but if it is, they may form part of the residue
that is left behind after dissolution.
In order to minimize the formation of insoluble carbonate
salts when mixing, the following suggestions may be helpful:
1. Add the salt to a full batch of water, rather than adding
water slowly to a large batch of salt. The latter allows
a greater time at much higher than natural seawater salinity,
which may tend to precipitate calcium and magnesium salts.
2. Stir the mixture vigorously as it is being dissolved.
3. If using a mix with a high initial pH, aerate the mixture
as well as stirring it. The aeration will reduce the pH.
Precipitates on Heaters and Pumps
The precipitate
that forms on heaters (Figure 2) and pumps is primarily calcium
carbonate. Natural seawater and reef aquarium water is
often supersaturated with calcium carbonate, but the precipitation
is kept at a very slow pace, primarily by magnesium ions (for
reasons described later in this article). Certain factors,
however, push the supersaturation even higher, and the "pressure"
to precipitate becomes too high for the magnesium to prevent
it. These factors include elevated calcium, alkalinity, pH,
and in the case of heaters and pumps, elevated
temperature. How these factors impact supersaturation
is detailed later in the article, but the following section
summarizes the effects.
Figure 2. Calcium carbonate can form thick deposits
on heaters.
It is the heat itself that helps drive this precipitation.
|
Chief among the factors causing precipitation are alkalinity
and pH.
While most aquarists would be unlikely to have in their aquaria
double the calcium level of natural seawater (more than 800
ppm), many folks have doubled its supersaturation level via
alkalinity and pH. With all other things being similar, for
example, an aquarium with a pH of 8.5 has twice the supersaturation
of one with a pH of 8.2, and an aquarium with a pH of 8.2
has twice the supersaturation of one with a pH of 7.9.
Alkalinity,
likewise, can be a big contributor to precipitation. Many
aquarists push alkalinity to elevated levels, approaching
or exceeding twice the naturally occurring levels (about 2.5
meq/L, or 7 dKH). That change can, in turn, double the supersaturation.
Elevated
temperature impacts calcium carbonate precipitation in
two ways: 1) by simply reducing the solubility of CaCO3
(which is more soluble at lower temperatures) and 2) by causing
the formation of additional carbonate from the bicarbonate
in solution. These effects are roughly similar in magnitude,
and are one of the reasons that pumps and heaters can be more
rapidly coated with calcium carbonate precipitate than other
objects in the same aquarium.
Another possible reason that pump impellers and heaters get
coated more rapidly than other surfaces is that such surfaces
may be less likely to become coated with a film of bacteria
that prevent calcium and carbonate from reaching the surface.
Heaters may become too hot for such bacterial films to form
properly, and pump impellers may have enough water motion
around them to wash away bacteria as soon as they begin to
attach. Exactly how large such effects are, however, is not
clear to me.
Low magnesium
can also contribute to precipitation because magnesium interferes
with the precipitation of calcium ions. Normally, magnesium
ions slip into spots where calcium would otherwise bind, altering
the surface's structure so that it no longer is a good seed
crystal for further calcium carbonate precipitation. Fewer
magnesium ions in solution makes this process less effective.
Finally, it appears that ferric
ion (Fe+++) may help
initiate the precipitation of calcium carbonate. Such ions
may be added directly in some products, and can be released
from iron-based phosphate binders such as Rowaphos, Phosban,
and Phosphate Killer.
Preventing Precipitates on Heaters
and Pumps
While the calcium carbonate that precipitates
onto heaters and pumps can be readily removed by soaking them
in acid (undiluted vinegar or diluted muriatic acid/hydrochloric
acid: 1 part acid added to 9 parts fresh water), it is often
easier to prevent the buildup in the first place. The following
actions can be taken if the aquarium has excessive precipitation
of calcium carbonate:
Note: actions 1, 3, and 4 may also make it harder
for calcifying organisms such as corals and coralline
algae to calcify, so they may grow more slowly if a significant
reduction is made in pH, calcium or alkalinity.
1. Reduce the overall pH.
The lower the better for this purpose, but I wouldn't go
below pH 7.8. Definitely target pH below pH 8.5, even at
the end of the light cycle.
2. Add high pH additives (limewater/kalkwasser
and high
pH two-part calcium and alkalinity additive systems,
especially) so that they mix quickly and do not enter pumps
or pass over heaters before mixing in well. Add them at
times of the day when low pH conditions exist (usually early
morning). Reduce these types of additives, if necessary.
3. Reduce the alkalinity. The lower the better for this
purpose, but I would not target levels below 2.5 meq/L (7
dKH). Definitely target alkalinity below 4 meq/L (11 dKH).
4. Reduce the calcium level. The lower the better for this
purpose, but I would not target the calcium below 380ppm.
Definitely target the calcium below 500 ppm.
5. Maintain an appropriate magnesium
level of 1250 -1350 ppm. Higher levels (up to, say, 1500
ppm) will help reduce precipitation further, and some aquarists
have resorted to this, but I do not know at what level magnesium
becomes toxic.
6. If you use an iron-based
phosphate binder, either situate it well upstream of
pumps and heaters or discontinue its use. Chelated iron
supplements (added to supply macroalgae with iron) are not
as likely, in my opinion, to cause precipitation, but may
still contribute to some degree.
7. Use pumps that do not get as hot internally (external
heat is not the concern here). I do not, however, have specific
advice on which pumps would be best in this regard. Plastic
impellers, while wonderful from a chemical resistance standpoint,
are poor heat conductors, so the heat that comes from pushing
against the water is not as rapidly dissipated as it would
be by impellers made from other materials.
8. Elevated phosphate
and organics
can reduce calcium carbonate precipitation. While I do not
recommend intentionally raising these for this purpose,
aquarists may find that if they reduce the levels of these
materials, that precipitation may become worse.
Precipitates Where Limewater is
Added
When
limewater is added to seawater, a cloudiness can form
almost immediately (similar to that in Figure 3, although
not usually that intense). This initial cloudiness is magnesium
hydroxide, Mg(OH)2,
and it forms when the water's pH rises into the low to middle
10's. Theoretical and experimental reasons for believing this
material to be Mg(OH)2
(and not magnesium carbonate or calcium carbonate,
for example) are given later in this article. As the limewater
is mixed in, the local pH around the particulates drops, and
as soon as it drops below pH 10, the magnesium hydroxide dissolves.
Figure 3. The transient cloud of magnesium hydroxide
that forms when high pH additives are added. In this
case, the alkalinity portion of B-ionic was added to
a fairly still portion of one of my reef aquaria.
|
If the limewater is not allowed to disperse rapidly enough,
meaning that the pH does not drop fairly quickly, additional
precipitates can form, especially calcium carbonate. Additionally,
if the limewater drips onto surfaces in contact with seawater
(such as the sides of a sump, Figure 4), bulk calcium carbonate
can form on those surfaces. This precipitation takes place
primarily because the limewater has pushed the CaCO3
supersaturation very high by converting much or all of the
bicarbonate into carbonate. Since the precipitation of calcium
carbonate can be slow to occur, rapid dispersal of the limewater
doesn't lead to much or any precipitation of calcium carbonate.
But if a region maintains high pH for long enough, calcium
carbonate will precipitate. How long this process takes depends
on the degree of supersaturation, but can be on the order
of minutes to hours.
Figure 4. When limewater drips onto surfaces,
such as the sides of a sump, precipitation of calcium
carbonate takes place. The off-white coloration probably
comes from metals such as iron binding to the calcium
carbonate surface in the place of calcium.
|
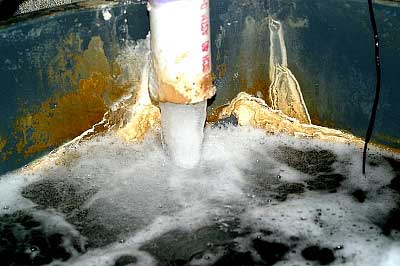 |
Precipitates When Carbonate Solutions
are Added
Many aquarists are familiar with the
cloudiness that forms when high pH two-part calcium and alkalinity
additive systems are added to marine aquaria. Figure
3 shows the initial cloud that forms, for example, when
the alkalinity part of B-ionic is added to a relatively low
flow reef aquarium. The initial cloud sinks and spreads out,
eventually dissolving. A similar phenomenon is not observed
when adding calcium or magnesium salts, but is observed when
adding sodium carbonate solutions.
This cloudiness is, at least in part, magnesium hydroxide
and is formed when hydroxide ions are added and the local
pH rises. Unlike the addition of limewater, which is unlikely
to form magnesium carbonate, this may, although I think it
unlikely. The reason it might form here is that the addition
of the carbonate ions may push the magnesium carbonate solubility
product above saturation. The precipitation of magnesium carbonate
can be kinetically slow, just as the precipitation of calcium
carbonate can be slow, and since this cloudiness forms instantly,
magnesium hydroxide is a much more likely candidate. However,
if the additive is not rapidly mixed in, or worse yet, if
solid globs of the initial precipitate settle out and are
very slow to dissolve, then conditions may be ripe for magnesium
carbonate (and calcium carbonate) to form.
In any case, any magnesium carbonate that does form will
probably dissolve later as the pH returns to normal reef aquarium
levels, so whether the initial cloudiness contains any magnesium
carbonate or not is not a critical issue. It does not contain
calcium carbonate if mixed in reasonably quickly (a couple
of minutes or less), as CaCO3
would not dissolve when mixed with seawater (and this material
is observed to dissolve).
Precipitates on Top of Limewater
The precipitate that forms on the
top of limewater
is calcium carbonate (Figure 5). Limewater
is high in calcium (about 800 ppm at saturation) and is very
high in pH (pH 12.54 at saturation), meaning that it contains
a lot of hydroxyl ions (OH-).
When carbon dioxide from the air encounters the water, it
hydrates to form carbonic acid:
(1)
CO2 + H2O →
H2CO3
Then, if the pH is above 11, as it is in limewater, the carbonic
acid equilibrates to form mostly carbonate:
(2)
H2CO3 + 2OH-
→
2H2O + CO3--
It is the carbonate that we are concerned with in the formation
of insoluble calcium carbonate, both on the surface of, and
inside, the limewater:
(3)
Ca++ + CO3--
→
CaCO3 (solid)
The result of this reaction is visually obvious. The calcium
carbonate can be seen as a solid crust on the limewater's
surface that has been exposed to the air for a day or two
(do not bother to remove this crust; it may actually be protecting
the underlying limewater from further penetration by carbon
dioxide). The formed solids also settle to the bottom of the
container, and can, in fact, form down inside it. Since solid
calcium carbonate is
not an especially useful calcium or alkalinity supplement,
this reaction has the effect of reducing the limewater's potency.
With sufficient exposure to air, such as by aeration
or vigorous agitation, this reaction can be driven to near
completion, with little calcium or hydroxide remaining in
solution.
Figure 5. A view of my limewater reservoir showing
a thin calcium carbonate crust on part of the liquid
surface, and heavy deposits of calcium carbonate, calcium
hydroxide, magnesium hydroxide, and other materials
on the bottom. I clean out the bottom of the reservoir
only once a year or so.
|
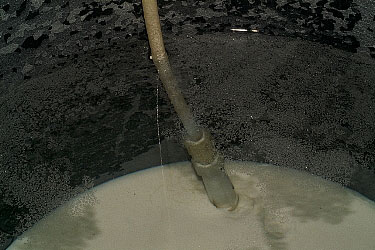 |
This reaction is the basis of the claims by many aquarists
that limewater must be protected from the air. It is also
the basis of the claim that limewater reactors (Nilsen reactors)
are to be preferred over delivery from still reservoirs of
limewater. Neither of these claims, however, stands up to
experimental scrutiny, as I showed in a previous
article.
Precipitates on Limewater Drip
Tips
Figure 6. Calcium carbonate that forms on the
end of the tubing where limewater drips into my sump.
|
The same reaction between limewater
and carbon dioxide that takes place on the surface of a limewater
reservoir (Figure 5) to form calcium carbonate
(described above) can also take place on a limewater drip
tip (Figure 6). Such tips rapidly become clogged, and need
periodic cleaning. This tip can be cleaned by soaking it in
acid (straight vinegar or diluted muriatic acid/hydrochloride
acid: 1 part acid added to 9 parts fresh water). One can also
periodically push a pencil or similar object through the crust
to ensure that the opening is large enough for limewater to
exit.
I once neglected to clean this tip on my system for too long,
and it completely sealed over. Without my knowledge, my Reef
Filler pump, though pumping away, was not delivering any top
off water. Only when my float switch shut down the main pumps
did I realize that there was a problem. Unfortunately, I made
a foolish mistake and poked a nail though the crust on the
tip without shutting down the pump. These pumps are actually
able to develop quite a bit of head pressure, and a fair amount
of milky limewater sprayed out over me (fortunately I had
glasses on, protecting my eyes)!
Some aquarists have suggested keeping the tip underwater
to avoid a reaction with atmospheric carbon dioxide. Unfortunately,
that is worse, because there is actually a lot of bicarbonate
and carbonate in marine aquarium water, and as this water
mixes with, and perhaps diffuses up the limewater tubing slightly,
an even more rapid precipitation of calcium carbonate will
occur, thus clogging the tip sooner.
Precipitates on the Bottom of
Limewater Reservoirs
The solids on the bottom of a limewater
reservoir (Figures 5 and 7) contain everything
that did not dissolve, or that dissolved and later precipitated
from solution. Such solids could contain magnesium hydroxide
and carbonate, calcium hydroxide and carbonate and a variety
of other impurities such as copper salts, alumina, silica,
etc.
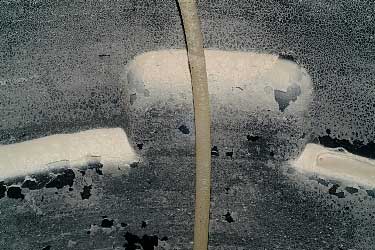 |
Figure 7. A view of the side of my limewater
reservoir after the water level has lowered. The precipitate
is most likely calcium carbonate formed when residual
limewater on the sides reacts with air, but may also
include solids that settled from the initial mixing,
and so may resemble the material found on the bottom
of the container (Figure 5). I can't recall ever cleaning
off the sides of this container.
|
In order to determine what is in these deposits, I tested
a sample of the white solid material that had been collecting
for months on the bottom of my limewater reservoir, and detailed
the results in a previous
article. I removed the white sludge along with some limewater.
The mixture of solid and liquid was acidified to dissolve
it, and it was tested for calcium, magnesium, and strontium.
The results are shown in Table 1. Only relative concentrations
are shown as no effort was made to dry the sample prior to
analysis, making absolute concentrations meaningless.
Table
1. Alkaline earth metals in limewater sludge.
|
Metal |
Relative
Concentration (by weight)
|
Enrichment
Relative to Solid Lime
|
Magnesium |
0.05
|
13.
|
Calcium |
1.00
|
1.00
|
Strontium |
0.00019
|
0.5
|
As anticipated, based on the very low solubility of magnesium
hydroxide and the high concentration of hydroxide ion in limewater,
the solid material on the bottom of the limewater is enriched
with magnesium. Relative to calcium, magnesium is enriched
by a factor of 13 in the sludge compared to the solid starting
quicklime. This magnesium may be present as both magnesium
hydroxide and magnesium carbonate, but because magnesium carbonate
is fairly soluble compared to calcium carbonate, it is most
likely that the primary magnesium salt is magnesium hydroxide.
It may also be mixed calcium and magnesium carbonates.
Interestingly, strontium is actually depleted by a factor
of two relative to solid starting quicklime, indicating that
it is less likely than calcium to end up on the bottom of
the reservoir. While strontium carbonate is somewhat less
soluble than calcium carbonate, the strontium concentration
in the limewater is so low that SrCO3
may not actually be saturated, so it may not precipitate at
all. The strontium that is there may simply be copreciptiated
with calcium carbonate.
The solids on the bottom of a limewater reservoir
or the residue left in a limewater reactor can also contain
other materials. Phosphate,
for example, would be insoluble in limewater, precipitating
as calcium phosphate. Many toxic metals, such as copper, are
also insoluble in the high pH of limewater, forming carbonates
or oxides. These metals can also bind directly to undissolved
lime or to calcium carbonate precipitates, as I showed in
a
previous article. In a sense, this precipitation can purify
the limewater so that in some cases it may be even purer than
the starting water or lime.
This purification is also seen in practice by many aquarists
who have noticed the solids on the bottom of their limewater
containers discolor, often to a bluish/green color suggesting
copper. For these reasons, I recommend that lime solids not
be dosed to aquaria when it is possible to avoid it. Letting
the limewater settle for a few hours to overnight will permit
most of the large particles to settle out, and whether it
looks clear at that point or not, it is likely fine to use.
In general, it is a good practice to leave residual solids
on the bottom of limewater reservoirs rather than cleaning
them out every time, as they may actually help purify the
water by these precipitation mechanisms. Once the solids discolor,
or have been collecting for 6-12 months, however, they should
be discarded.
Precipitates in Limewater Delivery
Tubing
Precipitates can also form inside
the tubing that delivers
limewater to the aquarium (Figure 8). Some of this material
will have been formed in the reservoir, and would therefore
have been carried into the tubing in particulate form. These
solids will be similar in composition to those found on the
bottom of the limewater reservoir (described in the previous
section). Some of this material, however, may form in
situ as carbon dioxide enters the limewater through the
walls of the tubing. Different materials have different permeability
toward carbon dioxide, and different thicknesses will also
alter its diffusion into the tubing. The diffusion can be
sufficient, however, that over the course of a year significant
amounts of calcium carbonate solids may accumulate in this
way, and may eventually clog the tubing. For this reason,
I flush acid through the system once a year or so to dissolve
this calcium carbonate buildup.
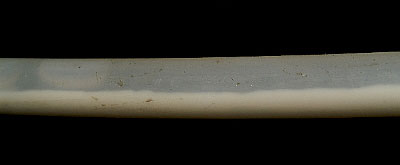 |
Figure 8. The settling of precipitated calcium
carbonate and possibly other materials in limewater
delivery tubing.
|
Precipitates from Overdosing
Limewater
When limewater is substantially overdosed,
the transient precipitation of magnesium hydroxide from normal
use may not be the only precipitate that forms. If the pH
becomes elevated and stays that way long enough, calcium carbonate
can precipitate throughout the water column. In such situations,
the entire aquarium can become very cloudy, looking almost
like skim milk (Figures 9 and 10). Such precipitation
events have the beneficial effect of lowering the pH and alkalinity
that were raised by the overdose, limiting the ongoing damage
that takes place. In many cases, there is no apparent harm
after a day or two, but in a few rare cases, when the overdose
was especially extensive, a tank crash can occur, killing
many organisms.
Figure 9. A reef aquarium experiencing a limewater
overdose, with calcium carbonate particles filling the
water column. This is the aquarium of Reef Central members
Ereefic and Klasikb.
|
The following important points should help in dealing with
a limewater overdose:
1. Don't panic! These overdoses do not usually cause a
tank to crash.
2. The primary concern is pH. If the pH is 8.6 or lower,
you need not do anything. If the pH is above 8.6, then reducing
the pH is the priority. Direct
addition of vinegar or soda water is a good way to accomplish
this goal. Either one mL of distilled white vinegar, or
six mL of soda water, per gallon of tank water will give
an initial pH drop of about 0.3 pH units. Add either to
a high flow area that is away from organisms (e.g., a sump).
3. Do not bother to measure calcium or alkalinity while
the tank is cloudy. The solid calcium carbonate particles
will dissolve in an alkalinity test, and all of the carbonate
in them will be counted as if it were in solution and part
of "alkalinity." The same may happen to some extent
with calcium tests. Wait until the water clears, and at
that point, alkalinity is more likely to be low than high.
Calcium will likely be mostly unchanged.
4. The particles themselves will typically settle out and
disappear from view over a period of 1-4 days. They do not
appear to cause long term detrimental effects to tank organisms.
5. Water changes are not necessarily beneficial
or needed in response to a limewater overdose.
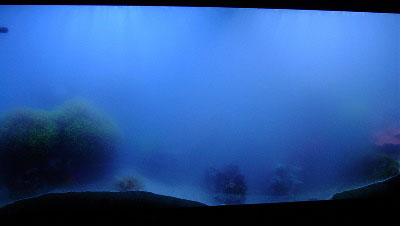 |
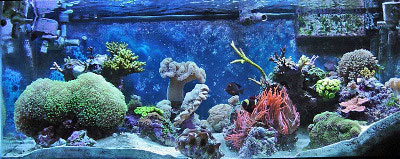 |
Figure 10. A reef aquarium experiencing a limewater
overdose (top), with calcium carbonate particles
filling the water column. The same aquarium the next
day was clear (similar to the bottom picture,
but taken a few days later). This is the aquarium of
Joe Robertson (Reef Central member Froggy).
|
Calcium Carbonate Precipitation:
Calcium Supersaturation Theory
In seawater, calcium
carbonate is supersaturated. In this context, that means
that given the right circumstances, it will precipitate as
solid calcium carbonate. Of course, under many other circumstances,
it doesn't do so, and the answer to the question of why that
happens is useful for aquarists to understand.
First, some definitions are in order. The equilibrium constant
expression for the dissolution of calcium carbonate is shown
below (equation 4):
(4)
K = [Ca++]x[CO3--]
Where K is a value determined by multiplying the concentration
of calcium by the concentration of carbonate. When K = Ksp*
(the solubility product constant in seawater at any given
temperature, pressure and salinity, which can be looked up
in a book), then the solution is said to be exactly saturated
(equation 5).
(5)
Ksp* = [Ca++]x[CO3--]
(at
saturation)
This relationship is often quantified using
the supersaturation parameter, which is symbolized as Ω:
(6)
Ω
= ([Ca++]x[CO3--]
)/ Ksp*
When Ω
= 1, the solution is exactly saturated. When Ω
exceeds one, it is supersaturated, and when Ω
is less than 1, the solution is undersaturated. The higher
the supersaturation, the more likely precipitation of CaCO3
will take place.
In normal seawater, Ω ~ 3 for
aragonite and Ω
~ 5 for calcite, though these values have been steadily dropping
as carbon dioxide has been added to the atmosphere, reducing
the seawater's pH. Aragonite and calcite are just different
crystalline forms of calcium carbonate. Calcite is slightly
more stable, and hence slightly less soluble, than aragonite
(i.e., has a lower Ksp*).
Organisms can precipitate both aragonite (pteropods
and corals) and calcite (foraminifera
and coccoliths),
but most of the precipitation in reef aquaria is aragonite
(although certain organisms such as abalone form both).
Reef aquaria often have higher alkalinity and calcium levels
than seawater, and hence are more supersaturated than seawater.
Alkalinity is a measure of the bicarbonate and carbonate in
solution. At a fixed pH, if the alkalinity is doubled, then
the carbonate will also be doubled. Since many aquarists keep
reef aquaria at alkalinity levels higher than natural seawater
levels (2.5 meq/L; 7 dKH), the supersaturation is often higher
than in the ocean.
The biggest driver of supersaturation in a reef aquarium,
however, might be pH. In aquaria with a high pH (such as many
aquaria using limewater) the supersaturation is much higher
than in seawater. At the same alkalinity, if you raise the
pH, you convert some of the bicarbonate into carbonate:
(7)
HCO3 - + OH-
→
CO3- - + H2O
At pH 8.2 and 25°C, only 15% of the total carbonate
and bicarbonate is present as carbonate. At pH 7.8, that value
drops to 7%. But as the pH is raised, that portion increases
to 50% at pH 8.93 and to 75% at pH 9.4. Consequently, as the
pH is raised at a fixed alkalinity, the concentration of carbonate
rises, thereby increasing the supersaturation of calcium carbonate.
Within the pH range of most reef tanks (up to about pH 9 or
so), the amount of carbonate present is approximately linear
with the pH because of the relationship seen in equation (7).
So if the pH rises from 7.5 to 8.5, there is approximately
a ten-fold increase in the carbonate concentration. From pH
8.0 to 8.5, the increase in carbonate is about threefold.
Above pH 9, the carbonate concentration continues to rise,
but more slowly, and it levels off above about pH 10 as there
is very little bicarbonate left at pH 10+ to convert into
carbonate.
Calcium Carbonate Precipitation:
Calcium, Alkalinity, and pH
Combining the various factors described
above, here are some combinations of calcium, alkalinity and
pH that have equal supersaturation (that is, equal propensity
to cause calcium carbonate precipitation):
Ω
= 1 (dissolution of aragonite takes place at all lower
values of these parameters)
|
pH
= 7.7
|
pH
= 8.2
|
Calcium
= 410 ppm
|
Calcium
= 340 ppm
|
Alkalinity
= 2.5 meq/L
|
Alkalinity
= 1.0 meq/L
|
Ω
= 3 (typical of normal seawater)
|
pH
= 8.2
|
pH
= 8.0
|
pH
= 8.4
|
Calcium
= 410 ppm
|
Calcium
= 410 ppm
|
Calcium
= 260 ppm
|
Alkalinity
= 2.5 meq/L
|
Alkalinity
= 4.0 meq/L
|
Alkalinity
= 2.5 meq/L
|
Ω
= 6 (non-biological precipitation is more likely)
|
pH
= 8.2
|
pH
= 8.2
|
Calcium
= 410 ppm
|
Calcium
= 820 ppm
|
Alkalinity
= 5.0 meq/L
|
Alkalinity
= 2.5 meq/L
|
|
|
pH
= 8.0
|
pH
= 8.7
|
Calcium
= 410 ppm
|
Calcium
= 410 ppm
|
Alkalinity
= 8.0 meq/L
|
Alkalinity
= 2.5 meq/L
|
|
|
pH
= 8.45
|
Calcium
= 410 ppm
|
Alkalinity
= 4.2 meq/L
|
How should we think about supersaturation? The higher it
is, the more likely it is that calcium carbonate will precipitate.
The reason for this is straightforward: if the "pressure"
to precipitate calcium carbonate becomes too high, certain
inhibiting processes (described below) will be overwhelmed,
and precipitation will take place.
If Ω
is not too high, some precipitation will take place before
the inhibiting mechanisms take control of the crystals' surface
and prevent further precipitation. This is the process that
happens in normal seawater. If Ω
is too high, a bigger precipitation event can take place before
being halted. In the worst cases, this can lead to a snowstorm
of calcium carbonate particulates throughout the tank. Such
snowstorms can occur, for example, when too much limewater
is added to the tank. In that case, the pH rises and converts
much of the bicarbonate to carbonate. Ω
is then driven to unstable levels, and a massive precipitation
event takes place.
Calcium Carbonate Precipitation:
Low Magnesium
Calcium carbonate is the most common
precipitate that forms in reef aquaria, and often forms on
pumps and heaters. As described above, calcium carbonate is
supersaturated in seawater and in nearly all reef aquarium
systems. In the ocean, calcium carbonate is supersaturated
by a factor of about three to five.
So why doesn't it immediately precipitate? There are several
reasons that calcium carbonate does not, under normal conditions,
rapidly precipitate, turning the water cloudy with a white
precipitate. The biggest factor is magnesium. Once a tiny
crystal of calcium carbonate begins to form, magnesium pushes
its way onto the calcium carbonate surface, and occupies a
site that would normally have been occupied by calcium. These
magnesium ions poison the surface against more accumulation
of calcium carbonate as it no longer looks like a crystal
of calcium carbonate to incoming calcium and carbonate ions,
and precipitation stops.
Magnesium is present in natural seawater at about 1280 ppm.
If magnesium is well below natural levels, then its effect
on calcium carbonate precipitation may be ineffective, allowing
precipitation to take place more rapidly than otherwise. Aquarists
experiencing such precipitation might consider testing for
magnesium, and supplementing if its level is not at least
1250 ppm. I usually recommend targeting magnesium at about
1250-1350 ppm. Exactly how low it needs to be before it begins
to allow calcium carbonate precipitation is not known exactly.
However, the relative impact of low magnesium in this context
depends on the relative pressure for calcium carbonate precipitation
to take place (which is shown in other sections of this article
to be determined primarily by the calcium concentration, alkalinity,
temperature and pH).
Calcium Carbonate Precipitation:
Elevated Temperature
For the reasons described above, many
aquarists tend to blame low magnesium levels for excessive
precipitation of calcium carbonate. In fact, while it is worth
testing when such precipitation is significant, low magnesium
is not the only way that such precipitation takes place.
It turns out that temperature also plays a significant role
in precipitation of calcium carbonate. This effect is noticeable
not so much via the aquarium's water temperature, although
that may have some impact, but most apparently, on unusually
warm objects in the aquarium, such as pumps and heaters. There
are two primary ways that warmer temperatures drive precipitation
of calcium carbonate. The first is simply by reducing the
calcium carbonate's solubility.
At S=35 (that is, normal seawater salinity) and 1 atmosphere
pressure, the Ksp* decreases
slightly as the temperature rises. Millero has published a
series of long equations for calculating Ksp*
for both aragonite and calcite.1
For aragonite, the log Ksp*
drops from -6.19 at 25°C, to -6.23 at 40°C to -6.44
at 80°C. In relative terms, the Ksp*
has fallen from 1 to 0.91 to 0.55 over this temperature range.
Likewise for calcite, the relative Ksp*
has changed from 1 to 0.96 to 0.73 over this range.
Consequently, if a reef aquarium has a supersaturation of
about 3 for aragonite and 5 for calcite at 25°C (typical
for seawater), then at 40°C the supersaturation has increased
to about 3.3 and 5.2, respectively. At 80°C this supersaturation
has increased to 5.4 and 6.8, respectively. Since the supersaturation
has increased, the likelihood of precipitation has increased,
and this increase is part of the explanation of why precipitation
takes place on the surface of heaters, where temperatures
can greatly exceed the bulk water temperature.
A second factor is the acidity of bicarbonate. Bicarbonate's
acidity increases with temperature, so the equilibrium for
equation 7 is pushed to the right as the temperature rises.
(7)
HCO3 - + OH-
→
CO3- - + H2O
In a previous article I worked through a variety of calculations
based on literary values for the acidity of HCO3-
as a function of temperature in seawater, and showed that
as temperature rose from 25°C to 40°C, the relative
concentration of carbonate increased by a factor of 1.45 as
equation 7 is pushed to the right. We also find, of course,
that the supersaturation of calcium carbonate (equation
6) has increased by a factor of 1.45 over this temperature
range. Running the same calculations for 80°C, we see
that carbonate concentration increases by a factor of 2.4x
compared to 25°C.
Combining these two ways that temperature impacts supersaturation
between 25 and 40°C for aragonite, we find a change from
Ω
= 3.0 to 3.3 due to solubility, and from 3.0 to 4.4 due to
the bicarbonate pKa shift. Together these effects yield a
supersaturation of 4.8 for aragonite.
Combining these two ways that temperature impacts supersaturation
between 25 and 80°C, we find a change from Ω
= 3.0 to 5.4 due to solubility, and from 3.0 to 7.2 due to
the bicarbonate pKa shift. Together these effects yield a
supersaturation of 13 for aragonite.
How do we put these supersaturation changes into perspective?
The following combinations of calcium and alkalinity have
the same supersaturation in seawater:
1. Normal seawater at 80°C
2. Seawater at 25°C with calcium raised to 1300 ppm
3. Seawater at 25°C with the alkalinity raised to 8.2
meq/L
It makes sense that all three of the situations above could
lead to precipitation of calcium carbonate, and that is exactly
what happens on the surfaces of hot objects in reef aquaria.
Magnesium Hydroxide
Magnesium hydroxide, Mg(OH)2,
can form in a variety of different ways in reef aquaria, but
it most often forms when the aquarium water's pH rises. At
higher pH there are more hydroxide ions (OH-)
, with about 10 times more OH-
for each 1 pH unit increase. The Mg(OH)2
that forms is quite insoluble, and it comes out of solution
as an amorphous white powder:
(8)
Mg++ + 2OH- →
Mg(OH)2 (precipitate)
In fact, this reaction is a well-known industrial process
for forming magnesium hydroxide that is later heated, driving
off water, to form magnesium oxide that has many industrial
uses. Most nonchemists know magnesium hydroxide as a suspension
of particles in water. Yes, it is Milk of Magnesia, sold at
the drugstore as a laxative. In that application, the solid
particles partially or completely dissolve, and the released
magnesium ions are poorly absorbed from the human intestines.
To maintain osmotic balance, water then enters the intestines,
giving a laxative effect as the stool is softened.
At normal reef aquarium pH, there is not enough hydroxide
to cause magnesium hydroxide to precipitate. At what pH does
it begin to precipitate? In a previous
article on metals in limewater, I showed how to calculate
the solubility of a variety of metals as a function of pH
in FRESH WATER. Figure 11 shows such data, and focusing on
magnesium, it can be seen that with a magnesium concentration
of 1280 ppm (the concentration in seawater), magnesium becomes
insoluble as magnesium hydroxide at pH values above about
pH 9.2 (that is, the solubility above pH 9.2 is below 1280
ppm, or below 1.3 x 103
ppm).
Figure 11. The solubility of magnesium in FRESH
water as a function of pH. The solubility of magnesium
drops below 1280 ppm (natural seawater levels) at pH
values above 9.2 (red line in graph), indicating that
magnesium hydroxide precipitates above this pH. The
much higher solubility of calcium/calcium hydroxide
is shown for comparison.
|
But is that also true in seawater? We can answer the question
both theoretically and experimentally. First, the theory.
The solubility of magnesium hydroxide is governed by a standard
solubility product equation:
(9)
K = [Mg++]x[OH-]x[OH-]
where K is called the solubility product, Mg++
stands for the concentration of free magnesium ions, and OH-
is the concentration of free hydroxyl ions. So it is readily
seen that as either the concentration of magnesium or the
pH rises, K increases.
The specific value for K, called the Ksp
or the solubility product constant, can be found in a book.
When the aquarium's K value exactly equals the Ksp,
the water is exactly saturated with magnesium hydroxide. When
K exceeds the Ksp, magnesium
hydroxide, it is likely to precipitate from solution. So,
we can calculate when K exceeds Ksp
in seawater since we know the pH (from a measurement), the
magnesium concentration (from a measurement or known values
for natural seawater) and the Ksp
(from a book).
It turns out that such a calculation is a little more complicated
than that, since not all of the magnesium ion present in seawater
is "free" magnesium. Rather, some is tied up interacting
with other ions. In seawater, it is known that magnesium concentrations
need to be adjusted downward in such calculations by a factor
of about 0.255 (with 0.255 being called the activity coefficient).
Consequently, a normal magnesium concentration (1280 ppm =
0.053 M) needs to be corrected to 0.0135 M.
Hydroxide ion is rarely measured directly, but is calculated
from the pH. We can calculate it from the known relationship:
(10)
[OH-] = Kw*/[H+]
Where Kw* is a constant
(for seawater at a given temperature) that can be looked up
in a book, and
(11)
[H+] = 10-pH
Like magnesium, the hydroxide and H+
ion concentrations must also be corrected with an activity
coefficient in seawater, and those values are known to be
about 0.236 and 0.688 respectively (in practice, however,
the value coming out of equation 11 for H+
is already corrected by the activity coefficient correction
for H+, so we need not do
that correction twice). So knowing the magnesium concentration,
the constants Ksp (= 1.2
x 10-17 when the concentrations are in molar units) and Kw*
(= 6.45 x 10-14 in molar units at 25°C) and the activity
coefficients, we can determine K as a function of pH (Table
2). As can be seen, K exceeds Ksp
when the pH is above about pH 9.4 (which is not especially
different from fresh water).
Table
2. K for magnesium hydroxide as a function of pH in
seawater at 25°C.
|
pH
|
K
|
8.2
|
7.7
x 10-14
|
8.5
|
3.0
x 10-13
|
8.8
|
1.2
x 10-12
|
9.1
|
4.8
x 10-12
|
9.4
|
1.9
x 10-11
|
9.7
|
7.7
x 10-11
|
10.0
|
3.0
x 10-10
|
10.3
|
1.2
x 10-9
|
10.6
|
4.8
x 10-9
|
10.9
|
1.9
x 10-8
|
Experimental Measurements of
Magnesium Hydroxide Formation
In order to substantiate the theoretical
calculations described above for magnesium hydroxide, I also
tested the precipitation of magnesium hydroxide at elevated
pH. Starting with fresh Instant Ocean artificial seawater,
I added dropwise a concentrated solution of sodium hydroxide
in fresh water. As each drop entered, a cloudy/white glob
formed that settled to the bottom. At first, each drop could
be fully dissolved with sufficient swirling. By the time the
pH of the solution reached the upper 9's, the glob was only
very slowly dissolving. By pH 10.4, the solution was very
cloudy, even after extensive swirling.
Taking this cloudy solution at pH 10.4, I added hydrochloric
acid to reduce the pH to 10.0. All of the cloudiness disappeared
within a few minutes. When set aside for 90 minutes, however,
a solid precipitate formed. Get the best personal care and skincare
products with Boots Sale.
Upon reducing the pH to 8.2 with
hydrochloric acid, this precipitate remained. It remained
undissolved for many days.
My conclusion is that the initial cloudiness is magnesium
hydroxide that rapidly forms and dissolves (not magnesium
carbonate, as described below). It appears somewhat more soluble
than the theoretical calculations above would suggest. That
is, it does not precipitate out until the pH is above 10,
and seems fully soluble at pH 10, while the calculations suggest
that the initial point of precipitation should be lower (mid
9's). This result appears to be because the magnesium hydroxide
that precipitates first is not the most stable form, and it
is the most stable form (the crystalline form brucite) that
is typically reported in Ksp values. The initial precipitate
is faster to form, but is more soluble.
The notion that there are different forms of magnesium hydroxide
that can form is supported in the chemical literature. One
group has reported, for example, that the initial precipitate
that forms when raising seawater's pH is not the most stable
form, that only more slowly is made as the initial precipitate
slowly converts into a less soluble crystal. They note: "In
the case of Mg(OH)2, a considerable
difference between the solubility of freshly precipitated
hydroxide and that of aged hydroxide was revealed."2
A second group reported similar findings: "Precipitated
Mg(OH)2
is at first more soluble than brucite, but in time its solubility
decreases to that of brucite."3
When sitting at high pH for more than a few minutes, calcium
carbonate can precipitate from these high pH solutions, as
was observed. Since seawater is supersaturated with respect
to calcium carbonate, even at pH 8.2, this material does not
dissolve on reducing the pH to 8.2, even after many days of
exposure.
Magnesium Carbonate Solubility
According to Millero in "Chemical
Oceanography,"1
natural seawater is undersaturated in magnesium carbonate1
(pure magnesium carbonate, not mixed calcium/magnesium carbonates)
by a factor of 27. When the pH of seawater is raised with
hydroxide ion (as by the addition of limewater or sodium hydroxide
solution), the only thing that could lead to magnesium carbonate
precipitation is the conversion of bicarbonate to carbonate,
raising the solubility product:
(7)
HCO3 - + OH-
→
CO3- - + H2O
(12)
K = [Mg++]x[CO3--]
This process, however, is limited by how much bicarbonate
can be converted into carbonate. At pH 8.2 in seawater, 15%
of the total carbonate plus bicarbonate is present as carbonate.
Even if all of the remaining bicarbonate were converted into
carbonate, the carbonate concentration would rise by only
5.7-fold. Consequently, the increase in pH is not enough to
cause magnesium carbonate to reach saturation and permit solid
magnesium carbonate precipitation. This fact is consistent
with the commercial process of forming magnesium hydroxide
(and not magnesium carbonate) by adding hydroxide ion to seawater.
Even artificial seawater with elevated carbonate alkalinity
does not contain enough carbonate to exceed saturation. For
example, an alkalinity of 5 meq/L (14 dKH) at pH 8.2 has double
the total bicarbonate and carbonate compared to natural seawater.
This doubling and the 5.7-fold increase from converting all
the bicarbonate to carbonate (2 x 5.7 = 11.4) still does not
attain the 27-fold increase in K that would be required to
reach the point of precipitate formation.
Consequently, I conclude that magnesium carbonate does not
form when hydroxide-containing additives (such as limewater)
are added to reef aquaria.
Can it form when carbonate salts are added? For example,
when sodium carbonate solutions are added as part of a two-part
additive system? Theoretically, yes. However, calcium carbonate
is kinetically slow to precipitate even when it can do so
(a well established fact that is experimentally reproduced
in the preceding section), and it is anticipated that the
kinetic issues that slow calcium carbonate precipitation (the
need to fit ions carefully into a crystalline structure) are
similar for magnesium carbonate (but not magnesium hydroxide,
which is amorphous) and will slow calcium carbonate precipitation
as well.
So I think it likely that magnesium carbonate
may form if carbonate salts are maintained at high concentration
for extended periods, but not likely when carbonate-containing
additives are well mixed into a reef aquarium within a couple
of minutes of their addition.
Summary
Many precipitates are formed in the
various processes that aquarists use to maintain reef aquaria.
Some of these may be beneficial, such as the solid material
that settles out of limewater, reducing the load of impurities
it delivers to the aquarium. Others are neither beneficial
nor detrimental, such as the initial cloudiness that forms
when high pH additives are added to reef aquaria. Others are
downright detrimental, such as solid calcium carbonate that
can clog pumps. The information provided in this article should
help aquarists understand what these are and why they form.
Using that information, aquarists may be able to better maintain
their reef aquaria without excessive worry over insignificant
issues.
Happy Reefing!
|