Beginnings
Although aquarists
often assume that research has been done on all types of marine
animals, in reality the body of information about most of
these creatures is vanishingly small. This is particularly
true of coral reef animals, about which what is not known
far outweighs what is. While coral reefs have been known as
long as someone has been around to fish on them, scientific
research on them has been a very recent endeavor, indeed.
The first ecological research on coral reefs was done in the
1920's but, strange as it may seem, very little additional
work was done over the next 30 or so years. Research on Caribbean
coral reefs really didn't start until the late 1950s, and
as recently as the 1970's the number of people actually out
in the field doing scientific investigations would not fill
a small meeting room. The reasons for this lack of research
revolve primarily around logistics, expenses and the development
of equipment. In general, the ecological sciences developed
in England, the United States, Canada and the northern European
countries. Coral reefs are distant from these countries, expensive
to visit and relatively difficult to study. For reasonable
ecological research to occur, the investigator needs to be
on the site for extended periods. Shallow water marine environments
of all sorts had to await the development of some sort of
equipment to allow the researcher to be on location for extended
periods. The necessary equipment in this case was SCUBA (an
acronym for Self-Contained Underwater Breathing Apparatus).
It wasn't until the late 1960's that a few scientists began
using scuba as a research tool. Even then, because of the
expense in getting to them, coral reef areas were not extensively
studied.
Underwater research is difficult in a number
of regards. Non-divers do not seem to comprehend the problems
inherent in moving in a viscous medium where visibility is
severely limited even at the best of times and where currents
and surge can make even taking notes difficult.
Additionally, because of the necessity of breathing pressurized
air, and of being under high pressure, the time on site is
very limited. In essence, the dollars per datum ratio is very
high; or, put another way, an underwater researcher doesn't
get much bang for the buck. Most in-depth ecological research
is done by graduate students as part of their, primarily,
doctoral research programs. Such programs are often designed
to last several years and provide a significant body of information.
Due to the nature of funding agencies, however, such studies
are often run on shoestring budgets. The bottom line is that
1) it has been very difficult for decent long-term underwater
water ecological research of any sort to occur, and 2) when
good projects are carried out, they are generally done in
close proximity to a marine laboratory. Most marine labs are
in temperate regions distant from coral reefs, and this is
where most marine ecological research occurs. The result is
that until, say, the last 15 years or so, most ecological
research carried out on coral reefs was of the nature of short-term
projects and even relatively little of those were done. That
result leads inescapably to the real bottom line which is:
we don't really know diddly about most coral reef animals.
Life History
The term "life
history" is a misnomer. What the term really means is
the generalized "story" of an average individual's
life. Probably the animal whose life history is best known
is the human. Broken down by geographical region and other
demographics, it is possible to predict with surprising accuracy
the life span, as well as the major life experiences, such
as the number of offspring, and when they occur, of the average
individual of many human populations. In the case of humans,
the driving force for the accumulation of such knowledge is
not the desire for "abstract knowledge," but rather
the desire to make a profit. On such knowledge is the insurance
industry built, and it is based to a great extent on studies
of human life history profiles extended to the greatest precision
possible. We also know the life histories of a large number
of other, mostly terrestrial, organisms. In these cases, biologists
have spent a great deal of time studying these animals' populations
and have noted when the animals are born, when they die, how
long they live, when they mate and so on. All of these data
can be massaged and manipulated statistically to provide a
good understanding of the essential experiences of an average
member of those species.
However, once we move out of the terrestrial
realm and into the oceanic environment, our ability to see
all of the relevant aspects of any given organism's life is
significantly obscured by a wall of water. Nonetheless, biologists
do know many aspects of a great many marine animals' life
histories; the majority of what has been written, however,
comes from investigations of animals undertaken from temperate
areas. By and large, when biologists discuss the life histories
of most tropical marine animals, they wave their arms and
generalize a lot. In essence what they do is take what is
known about similar temperate species, "rub a little
biological 'funk' on it" and warble a prognostication
about what they think will happen or what is happening in
the tropical environment. Depending upon the organism in question,
the frequency of the arm waving may generate quite a nice
hum. What it doesn't do, however, is actually describe what
is occurring with regard to the tropical organism being discussed.
That doesn't take arm waving or prognostication. It takes
good, down to earth (or ocean bottom), tedious, long-term,
expensive and dedicated research. Frankly, very little of
that has been done on any tropical species - and it has been
completed for precious few temperate ones.
The Story
In this article
I summarize the results of a series of research projects concerning
a temperate octocoral, the sea pen, Ptilosarcus gurneyi.
This species has also been known as Ptilosarcus quadrangulare,
Leioptilus quadrangulare, Leioptilus guerneyi
and Ptilosarcus quadrangularis, but these names have
long been considered to be junior synonyms. Nonetheless, they
still turn up from time to time, particularly in comments
on the internet. The basic research on which this article
is based was reported in two papers (Chia and Crawford, 1973;
Birkeland, 1974), but in addition, there is a significant
amount of my own hitherto unpublished research. For ease of
readability, I will not cite either reference again. If the
reader is interested in more detail, please read these articles
or contact me directly. In total, this information represents
several thousand person-hours of observational time. As far
as I know, no similar body of knowledge exists for any tropical
octocoral. I hope, however, that this example will elicit
some appreciation for the life history of at least one type
of coral, and also perhaps the types of data that are needed
to be able to cogently discuss these animals.
|
Figure 1. A mature Ptilosarcus gurneyi
individual extended about 50 cm out of the sediment.
The primary polyp consists of the base extending into
the sediment, and the rachis or stalk extending up between
the "leaves." The gastrozooids or feeding
polyps are found on the outside edges of the leaves,
and the siphonozooids or pumping polyps are found in
the orange regions on the sides of the rachis. The "warts"
on the leaves are caused by a parasitic isopod living
inside the pen's tissues.
|
Figure 2. A portion of a sea pen bed in the central
Puget Sound region; the depth was about 15 m. Water
flow was from the left, and the foreground field of
view is about one meter (3.3 feet) across.
|
Sea pens are octocorals. Taxonomically,
they are placed in the Order Pennatulacea
of the Subclass Octocorallia (= Alcyonaria), in the Class
Anthozoa of the Phylum Cnidaria. All of this jargon means
they are animals whose major body parts consist of modified
polyps. All are sessile, living in unconsolidated ocean bottom
sediments. They are not fastened to the substrate and at least
some sea pens are capable of significant locomotion. Being
octocorallians, they have polyps that show an octamerous or
eight-fold symmetry. The most obvious manifestation of this
symmetry is the presence of eight tentacles around the mouth
of the feeding polyps, or gastrozooids. These tentacles each
have small side branches, a condition referred to as "pinnate
branching." The adult sea pen's body is considered to
be comprised of modifications of three types of polyps. The
base and central stalk region, or rachis, is considered to
be the modified original polyp. The gastrozooids are found
either on the surface of the rachis, or on lateral extensions
from the rachis. Depending on the species and its adult size,
there may be from about ten to many thousand gastrozooids.
Embedded in the rachis' surface are the siphonozooids, which
are modified polyps lacking tentacles. These structures consist
of a "mouth" which is lined with microscopic, beating,
hair-like projections called "cilia." Siphonozooids
pump water into the colony's body. The colony has quite an
intricate system of channels that allow the movement of water
and nutrients throughout the body. The force necessary for
water movement comes from muscular contraction of the body
and the beating of the cilia lining the water channels.
Figure 3. The edges of a mature sea pen's leaves,
showing their numerous gastrozooids. The leaves are
oriented into the current, and can generate hydrodynamic
lift. When the animals are disturbed, they may orient
into the current, inflate, climb out of the sediment
and drift away in the current.
|
Figure 4. A photograph of a sea pen's rachis,
showing the siphonozooid region. Siphonozooids are basically
polyps without tentacles that pump water into the pen.
Note the small stenothoid amphipod found on the pen's
surface. These harmless animals are related to some
of the minute amphipods that are sometimes found on
aquarium corals.
|
Sea pens are like the late comic, Rodney
Dangerfield, in that they "don't get no respect."
They are often largely ignored in invertebrate zoology classes,
and hardly discussed at all in many marine biology classes.
Nonetheless, they are surprisingly abundant and, in fact,
may be the dominant cnidarians over most of the earth's surface
in areas where stony corals, other octocorals and most sea
anemones are essentially absent - the deep sea abyssal plain.
This is Earth's largest ecosystem and much of it is characterized
by the presence of vast fields of sea pens. I doubt anyone
has made the calculations, but I suspect that it would be
a sure bet to say that the biomass of sea pen living tissue
exceeds that of all other benthic cnidarians combined.
Figure 5. A small sea pen that had been drifting
across the bottom.
|
Ptilosarcus gurneyi (Gray, 1860), the subject of this
article, is found throughout the Northeastern Pacific from
Alaska to Southern California. Fully-expanded, large, adult
individuals may extend for about 60 cm (2 feet) out of the
sediments, with their base extending into the sediments another
15 to 30 cm (6 to 12 inches). Fully contracted, these same
large adults will be about 15 to 20 cm (6 to 8 inches) long.
Their body's color ranges from a pale cream to a deep orange-red.
Their body's morphology may bring to mind a fat, carrot-colored
quill pen. Internally, they contain a single large calcareous
and proteinaceous object called a style.
The style's size is related to the sea pen's age and
may be used to obtain ages of individuals in a population.
Ptilosarcus gurneyi are found in shallow waters, ranging
from the lowest intertidal zone to depths of about 500 feet,
but are most abundant in shallow waters. And, they may be
amazingly abundant. In the Puget Sound areas studied
by Birkeland, the number of sea pens averaged up to about
23 pens per square meter, with an average of about 8 of these
being three years old or older. These sea pen beds typically
range to depths in excess of 50 m, and extend laterally for
dozens of kilometers, interrupted only by physical features
such as scouring river currents, dredged harbors and the like.
Although these beds are discontinuous, because of the area's
currents they are not isolated from adjacent beds. Larvae
are dispersed throughout the region, and even adult pens may
move great distances. The adults can crawl up out of the sediment,
inflate with water and drift along in the currents, much like
an underwater balloon.
Figure 6. A sea pen photographed in the field
a day or two prior to spawning. Note the eggs in the
bodies of the gastrozooids. The extended nature of the
gastrozooids is visible in the image. Their guts extend
through the leaves into the rachis where they meet and
fuse with a large internal canal system that extends
throughout the animal. The eggs are about 0.600 mm in
diameter.
|
The sea pens have a cyclic behavioral pattern during which
they inflate, feed and then deflate. They may do this several
times a day, and the rhythm appears more-or-less unrelated
to feeding or available foods. Birkeland found that at any
one time, only about 26% of the pens were exposed, with the
rest being buried in the sediments. They completely bury into
the sediments, often to a depth of 30 cm (1 foot) or more.
The sea pens totally dominate and structure these communities.
Very few other macroscopic animals live in the sediments of
sea pen beds, possibly due to the continuous bioturbation
resulting from the sea pen's movement.
As might be expected, the sheer mass of sea pen flesh in
these areas is a resource that has not "gone unnoticed"
by predators. In fact, an amazing variety of predators have
become adapted and, in some cases, totally dependent upon
the sea pens. In many respects, Ptilosarcus gurneyi
in sea pen beds fill an ecological position similar to the
huge herds of bison that used to occupy the American Great
Plains, or to the grazing animals of the Serengeti. In each
of these cases, whole food webs were built upon the basic
keystone resource species.
Sea pens are suspension-feeding animals. They feed on small
organic particulate material, larvae and other small zooplankton.
In turn, they are fed upon by a wide variety of benthic predators.
When they are small, they are eaten by several species of
nudibranchs. As they grow, they become too large for the smaller
of the nudibranchs, although other larger ones may still eat
them. As the pens get larger, however, they become prey for
several species of sea stars. The top predator in the system
is yet another sea star, which
preys on those stars.
Figure 7. Evidence of incomplete or attempted
predation; in both cases these partially eaten sea pens
probably were attacked by nudibranchs.
|
Figure 8. The Predators.
These nudibranchs primarily eat the smallest pens and
become common in sea pen beds shortly after the juveniles
settle and metamorphose out of the plankton. Left:
Phidiana crassicornis. Right: Flabellina
fusca. Both nudibranchs are small, reaching lengths
that do not exceed about 4 cm (1.6 inches). These species
are responsible for the deaths, on average, of upwards
of 200 sea pens per square meter, per year.
|
Figure 10. The Predators.
These two large nudibranchs, Tritonia diomedia
(left) reaching lengths of 15 cm (6 inches) and
Tokuina tetraquetra (right) reaching lengths
in excess of 30 cm (12 inches), also eat sea pens. Although
Tritonia is common in sea pen beds, Tokuina
is not, and tends to eat isolated sea pens growing in
sediment pockets in rocks. These nudibranchs can eat
the largest sea pens without any difficulty whatsoever.
|
Figure 11. The Predators.
These images show Armina californica (left),
probably the most abundant nudibranch predator that
eats Ptilosarcus. Although capable of eating
a pen alone, these animals seem to be gregarious feeders
and are more often seen clustered over the remains of
their prey (right). These nudibranchs can reach lengths
of about 10 cm (4 inches) long, and will eat all sizes
of pens.
|
Figure 12. The Predators.
In the areas of the sea pen beds, about 50% of the food
items eaten by the rosy sunstar, Crossaster papposus,
are sea pens.
|
Figure 13. The Predators.
In the areas of the sea pen beds, about 98% of the food
items eaten by the leather star, Dermasterias imbricate,
are sea pens.
|
Figure 14. The Predators.
The vermilion sea star, Mediaster aequalis, is
a predator of sea pens, among other things. About 38%
of its diet in sea pen beds consists of the pens. Here
a sea star is hanging on to a pen that is expanding
and trying to dislodge it. Pens bury into the sediments,
and if stimulated the pen will sometimes inflate, expand
and attempt to dislodge the predator. Note that the
pen has lifted the sea star totally off the substrate.
|
Figure 15. The Predators.
The spiny sea star, Hippasteria spinosa, eats
only sea pens. It takes a full-grown star about four
to five days to eat a fully-grown sea pen.
|
Figure 16. The Predators.
The "top dog" in these ecosystems is the morning
sun star, Solaster dawsoni, here shown eating
a Stimpson's sun star, Solaster stimpsoni, which
it has in a literal death grip. Although relatively
rare in the sea pen beds, the morning sun stars can
and will eat all other sea stars that they come across.
As nothing eats them, they are the top predators in
the system.
|
Figure 17. The Predators.
The end result of all the predators' effects is the
style.
|
In the Water
In the first week following the spring
equinox, Ptilosarcus gurneyi generally spawns. In the
laboratory during that time, sunlight hitting a tank of gravid
sea pens that have been shaded will induce spawning. The eggs
are large, about 600 µm across, and a mature female
colony will produce upwards of 200,000 of them. Fertilization
occurs in the water column. Embryonic development occurs over
about three days at 12º C, and results in a football
shaped mobile, but non-feeding (lecithotrophic), planula larva
about 1 mm long. The planulae begin to swim to the bottom
of containers and, by inference, to the ocean's bottom by
the time the larvae are about five days old. They touch their
anterior end to the sediments, and if the sediments are coarse
sand, with particle sizes in the 0.250 to 0.500 µm range,
they settle into that sediment and begin to metamorphose into
small sea pens. If appropriate sediments are not available,
settlement and metamorphosis may be delayed for as much as
a month. These pens are found in areas dominated by vigorous
tidal flows and offshore currents. In a month, the larvae
could disperse over great distances.
|
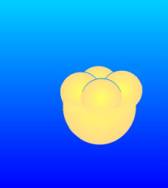 |
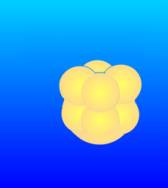 |
Figure 18. All of the drawings in this
article were done from living embryos and larvae of
developing Ptilosarcus gurneyi. Left:
The newly released ovum. Center: Embryo at about
the beginning of the 4-celled stage; about four hours
old. Cleavage is superficial and doesn't extend through
the embryo. Right: Embryo between the 4- and
8-celled stages, about six hours after fertilization.
|
Figure 19. Ptilosarcus gurneyi. Left:
The 8-celled stage, about the same diameter as a newly
spawned egg; about seven hours after fertilization.
Center: Embryo at the 16-celled stage; about
eight hours old. Right: Embryo at the blastula
stage, about twelve hours old.
|
Figure 20. Ptilosarcus gurneyi. Gastrula
stages; individual cells no longer visible. Left:
Early gastrula, irregularly shaped, about 24 hours old.
Center: Gastrula, about 36 hours old. Right:
Late Gastrula, the embryo has become ciliated and is
swimming; two days old.
|
Figure 21. Ptilosarcus gurneyi. Left:
Early planula, about three days old. Right: Planula
larva at four days old.
|
Figure 23. Ptilosarcus gurneyi. Left:
Planula at five days. Center: Planula, settling,
about six days. Right: Settled larva undergoing
metamorphosis into a juvenile sea pen, about eight days
of age. The animal is capable of contraction and elongation.
|
Once metamorphosis begins, development into a feeding individual
is rapid, with the first polyp having functional tentacles
within two weeks of spawning. Active feeding begins soon thereafter
and growth may be relatively rapid. Once the sea pens have
settled, they become potential prey for many predators, and
this predation pressure is extreme. Small sea pens are eaten
at the rate of about 200 per year per square meter by small
nudibranchs. If they can survive this period, they become
large enough to avoid becoming prey for the smallest of the
nudibranchs, but they become food for sea stars. Sea pens
grow at a relatively constant rate, reaching sexual maturity
when they are about 24 cm (9. 4 inches) tall and five years
old. Both growth and predation rates are steady; there is
no cessation of growth upon reaching an "adult size."
As with many cnidarians, there is no old age or senescence
demonstrable in these animals. Nonetheless, they don't live
forever. In essence, their life is an exercise in "beating
the odds," and as in all such cases, the "odds"
in this case, the odds of being eaten by a predator, are too
great for indefinite success. The life expectancy of an individual
sea pen in the Puget Sound areas appears to be about 14 to
15 years. Older animals may be found occasionally but, if
so, they are very rare. Predators aren't the only source of
mortality in sea pens, nor are sea pens immune from parasites,
which may influence their growth and survival; however, these
factors remain unstudied.
|
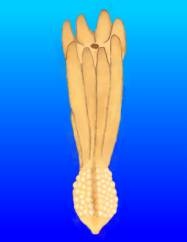 |
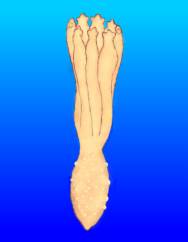 |
Figure 24. Drawings, done from life, of
a developing Ptilosarcus gurneyi. Larva undergoing
metamorphosis. Left: 11 days old. Center:
13 days old; tentacles clearly evident; bumps on body's
surface are of unknown function, spicules are not present;
mouth is open and a gullet or gut is evident. Right:
15 days old; note the development of the tentacles'
first pinnae.
|
Figure 25. Drawings, done from life, of
a developing Ptilosarcus gurneyi. Two individuals
about three weeks old. Spicules are evident in the body's
wall. They have one complete feeding polyp, the primary
polyp, and siphonozooids on the stalk. The animals were
actively feeding at this time and were about 3.5 mm
long. The extent of the visible gut is shown in the
individual on the right. These individuals were
settled and growing in coarse sand. At this point, I
terminated this growth series and transplanted the juveniles
to a sea pen bed in one of my research study sites.
|
Figure 26. A juvenile sea pen, photographed
in nature; depth about 15 m. The animal is about one
year old. It was between one and two centimeters (about
0.4 to 0.8 inches) high. Note the gastrozooids, and
the internal calcareous style (visible as a white band).
|
Figure 27. A juvenile sea pen, photographed
in nature; depth about 15 m. This animal is about two
years old. It was about 5 centimeters (about 2 inches)
high. Note the gastrozooids, the siphonozooids (visible
as bumps on the stalk) and the developing leaves.
|
Figure 28. An older juvenile sea pen,
probably about four years old, about 15 cm (6 inches)
high. The internal style that can be used to determine
the animal's age is clearly visible as a white internal
stripe.
|
Figure 29. The Green Disease. As if predation
weren't enough, sea pens are subjected to other maladies
as well. The green coloration in the pens above is caused
by an endoparasitic green alga, which appears to kill
the pens, albeit taking several years to do so.
|
Figure 30. Not all Ptilsosarcus gurneyi
in the Northeastern Pacific are found in dense beds.
They may be found in many other habitats where sand
pockets of the appropriate type occur. The anemones
to the left are Urticina piscivora, and are about
30 cm (12 inches) across the oral disk.
|
During their lifetime, these pens probably reproduce about
ten times. If the average number of eggs produced by one pen
is 200,000, then each female will produce about 2,000,000
eggs over her life span. If the populations are assumed to
be constant, neither shrinking nor growing, then over the
female's life span she must spawn only enough eggs to replace
herself and her mate, indicating the odds of survival to successful
reproduction by any given Ptilosarcus gurneyi individual
in the Puget Sound region is about 1,000,000 to 1. Although
those odds seem pretty slim, they are significantly greater
than many other marine animals such as pelagic fishes.
Figure 30. Juvenile Ptilosarcus
settle in dense assemblages; they have to, to be able
to withstand the predation pressure from the array of
predators that feed upon them.
|
Figure 30. Just to show they exist, here
is an image of an unidentified tropical sea pen, probably
a species of Veretillum, courtesy of Eric Borneman,
taken at Karang Koromaha Atoll, Sulawesi. His description
of taking the picture says all that is necessary about
underwater research, "[The picture] is not the
greatest, I was clutching onto the bottom in a raging
current at night on an outgoing tide out of an atoll,
and its not easy to take the perfect shot when rocks
are literally tumbling by and sea cucumbers are rolling
by and sand is making vortices on the bottom, but here
it is. I was in this channel between large bommies,
and there was nothing to grab onto where all the sea
pens had unfurled; maybe 3m of water, and I kept trying
to dig into the sand but just got blown away. Finally,
I found a nub of rock sticking out of the sand, latched
on, and took the shot with one hand."
|
To Take Home
Although temperate sea pens are not
likely to be an animal of choice for most reef aquarists,
tropical sea pens of several genera are, at least, moderately
commonly available to aquarists. For those interested in aquarium
husbandry, some things may be taken to heart from my tale
of sea pen life and, mostly, death. First, despite their apparent
simplicity, these are hardy animals capable of surviving much
environmental perturbation; unless eaten, they have the potential
to live a long time. Second, they are mobile and capable of
movement, and will leave areas that are not to their liking.
In a marine aquarium, tropical sea pens will have to be provided
with a deep sand bed - really deep - 30 cm (1 foot) or more
- to allow their innate activity patterns to be expressed.
Additionally, the appropriate physical habitat conditions
such as current regimes, temperature and salinity must be
matched to natural situations. Third, sea pens lack zooxanthellae,
yet they have a rapid growth rate. Consequently, they need
to be fed. They eat small zooplankton; organisms much larger
than newly hatched Artemia are likely to be too large
for them. They probably will not eat much phytoplankton unless
it is added as a dead suspension of clumped cells. Fourth,
sexual maturity is likely to be reached when the animals are
significantly less than full-sized. Eggs, at least, will be
quite noticeable as they develop in the "leaves"
below each gastrozooid. Sperm may be difficult to see, but
may be noticeable in similar bodily regions as white patches.
Given the number of eggs released by even a small individual,
it is likely that spawning events would significantly foul
a small aquarium. If eggs are seen developing in a "pet"
sea pen, then the aquarist must plan for the upcoming blessed
event. Finally, these are captivating and strikingly beautiful
animals. Additionally, they show activity patterns totally
lacking in the "colored sticks" kept by many aquarists.
If the hobbyist is willing to accommodate their specific needs,
primarily by providing a deep sand habitat for them to bury
into, and an appropriate diet, they likely will thrive.
If you have any questions
about this article, please visit my author forum
on Reef Central.
References:
Birkeland, C. 1974. Interactions between a sea pen and seven
of its predators. Ecological Monographs. 44: 211-232.
Chia, F. S. and B. J. Crawford. 1973. Some observations on
gametogenesis, larval development and substratum selection
of the sea pen Ptilosarcus gurneyi. Marine Biology.
23: 73-82.
Kozloff, E. N. 1990. Invertebrates. Saunders College
Publishing. Philadelphia. 866 pp.
Ruppert, E. E, R. S. Fox, and R. D. Barnes. 2003. Invertebrate
Zoology, A Functional Evolutionary Approach. 7th
Ed. Brooks/Cole-Thomson Learning. Belmont, CA. xvii +963 pp.+
I1-I 26pp.
|